Optimal control (OC) theory, through the implementation of numerical algorithms, is a powerful RF pulse design tool for NMR. It provides a solid theoretical framework to compute efficient RF pulses for a large variety of applications, while taking into account experimental constraints such as B0 or B1 field inhomogeneities, minimal pulse duration and power requirements.
The basic principle of this approach is to iteratively converge towards the optimal RF pulse that brings the magnetization into a pre-defined state, leading to the acquisition of a NMR signal with the desired characteristics.
Three main applications are currently being investigated:
- Contrast optimization: optimal contrast preparation sequences are designed to obtain non-trivial contrasts between structures of interest, based on their relaxation time difference (e.g. short-T2 enhancement).
- Magnetization phase control: OC RF pulses can accurately, and with a high degree of flexibility, encode into the magnetization phase various isochromat characteristics (e.g. motion in the context of MRE, resonance frequency).
- Robust and energy efficient excitation: OC RF pulses robust to inhomogeneous RF transmission can be computed, with a significantly lower energy deposition than adiabatic pulses, which is of interest in the context of SAR reduction. Low-energy excitation is also investigated in the context of low gyromagnetic ratio nucleus.
X-Nuclei
Preclinical (PILoT with the new 11.7T) and clinical (PRISM/CHUSE) platforms, with which the team works closely, are equiped with the necessary instrumentation to investigate other nuclei (besides hydrogen) such as 31P, 13C, 23Na. Developments of dedicated acquisition strategies for a given nucleus target applications in muscles and the digestive tract. For example:
- 13C and 31P in natural abundance provide, in both cases, valuable insight about the glucose and energy metabolism
- 23Na and 31P are involved in cellular homeostasis and energy metabolism notably through oxidative phosphorylation and the Na+/K+-ATPase pump.
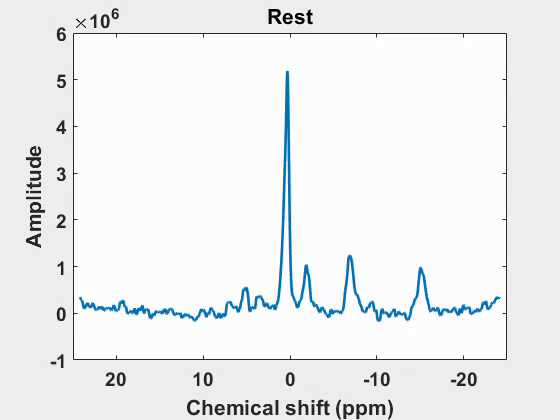
The assessment of energetic metabolism via 31P spectroscopy can be done with non-localized spectroscopy , single voxel selection spectroscopy and Magnetic Resonance Spectroscopic Imaging (MRSI). In clinical practice, mostly non localized 31P spectroscopy is do-ne, preventing metabolic information from different individual muscles to be measured. The use of localized 31P spectroscopy would enable to access spatially resolved information and motivate the development of new home made sequences integrating the most advanced technical developments. A fast MRSI acquisition method has thus been developed involving a non-cartesian k-space Sampling (spiral sampling, cf ), coupled to a smart under-sampling of the temporal dimension, exploiting a priori known spectral support and a least-square estimation for signal reconstruction.
This method has been validated using simulations, and implemented in a MR scanner, optimized and then tested in vivo on the calf muscle for 1H and 31P MRSI applications. A fast MRSI acquisition method has thus been developed involving a non-cartesian k-space Sampling (spiral sampling), coupled to a smart under-sampling of the temporal dimension, exploiting a priori known spectral support and a least-square estimation for signal reconstruction. This method has been validated using simulations, and implemented in a MR scanner, optimized and then tested in vivo on the calf muscle for 1H and 31P MRSI applications.
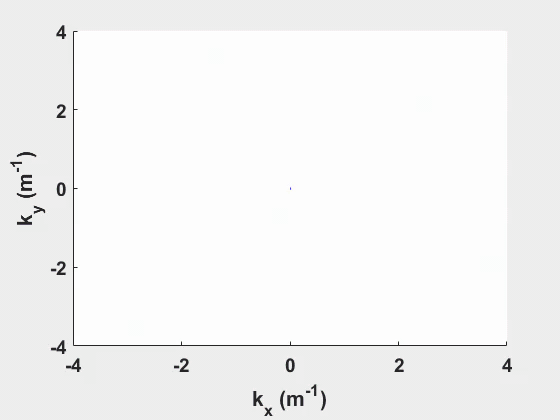
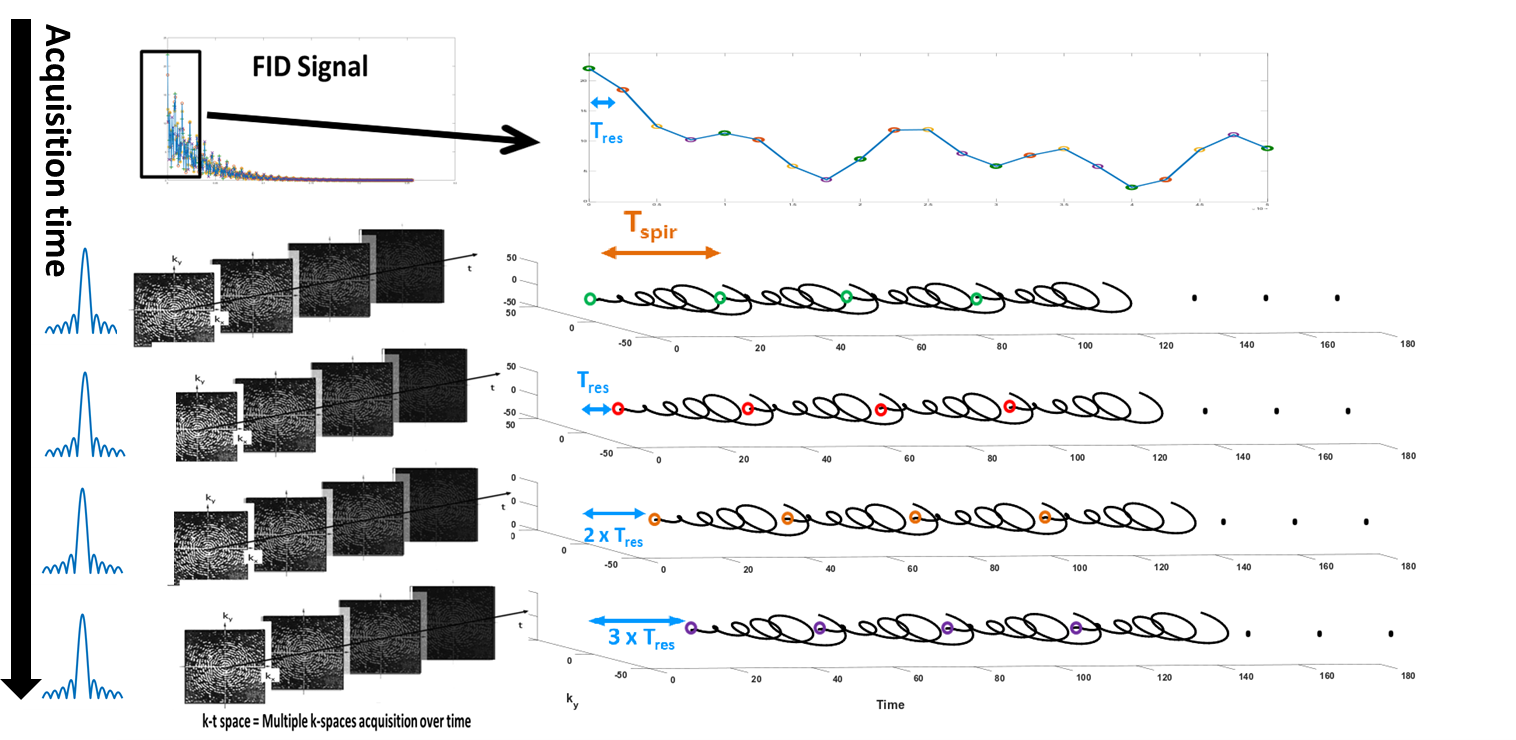